The severity of the bacterial antibiotic resistance phenomenon is growing, creating a major public health problem. One possible alternative to antibiotics involves the use of phages, or viruses that infect bacteria. However, a recent study has demonstrated that one of the principal resistance mechanisms used by bacteria against antibiotics is also used against phages. This work highlights the importance of formulating additional strategies to tackle antibiotic resistance and paves the way for developing biotechnological tools.
Bacteria first emerged on Earth 3.5 to 4 billion years ago, quickly developing adaptation mechanisms allowing them to survive in the changing and sometimes hostile environments that have been a recurrent feature of our planet throughout its history. They are able to use various processes to adapt to their surroundings, including occasional and random modifications to their genetic material (mutations), an aptitude for acquiring genes from their environment or those originating from other microorganisms (horizontal gene transfer), and an ability to rearrange their genome by displacing mobile DNA sequences within it.
Integrons – an adaptive tool for resisting antibiotics
This ability to adapt is now allowing more and more bacteria to develop antibiotic resistance. As a result, antibiotics, which have been used in mainstream medicine since the mid-20th century to tackle bacterial infections, are proving less and less effective. This means that it may become much more difficult to treat minor illnesses in future. If no action is taken, WHO estimates that antibiotic-resistant bacterial infections could lead to the deaths of 10 million people annually throughout the world by 2050.
"In some groups of bacteria, integrons are the main vectors of antibiotic resistance," explains Didier Mazel, Head of the Bacterial Genome Plasticity Unit at the Institut Pasteur. Integrons are like "a genetic toolbox," adds Céline Loot, a CNRS scientist in the same research unit. This toolbox contains:
- a gene coding for an integrase (IntI), an enzyme that cleaves DNA in specific locations
- a promoter (Pc), a small DNA sequence located upstream of a gene and essential for its expression
- gene cassettes, mobile DNA sequences generally containing one gene and ending in specific attC sites
- an integration site named attI
Bacteria express integrase in certain conditions involving stress, for example if antibiotics are present. This protein cleaves the gene cassette at the attC sites enabling its excision. The excised cassette is then integrated at the attI site, near the promoter Pc, thus enabling expression of the gene carried by this cassette. This rearranges the bacterial genome, and the bacterium acquires the characteristic associated with the newly expressed gene.
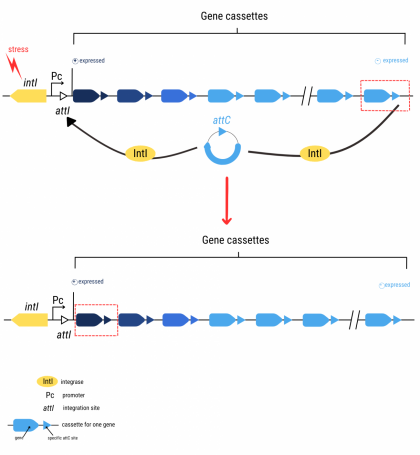
While it is known that antibiotic resistance genes are present in cassettes, many of these cassettes carry genes whose functions are unknown. Scientists at the Institut Pasteur, CNRS, Université Paris Cité and University of Montreal were keen to learn more about this. Their work revealed that a notable percentage of these cassettes contain systems defending against phages.
Same approach taken to fighting phages and antibiotics
To provide a little background on the topic, phages are viruses that only infect bacteria. Though considered a promising avenue of research for fighting bacterial infections in the early 20th century, they were superseded by the mass adoption of antibiotics in the late 1940s and early 1950s, with work on these viruses more or less put on hold. However, due to increasing antibiotic resistance, there has been a resurgence in interest in recent years in these viruses that could be used as substitute therapies against pathogenic bacteria.
Now, for the first time, scientists have demonstrated that integrons are also involved in phage resistance. In other words, bacteria use the same adaptive weapons against antibiotics and the viruses that infect them! The extent of this phenomenon exceeded the study authors' expectations. "We estimate that at least 10% of integron cassettes code for anti-phage systems. We had no idea that this percentage would be so high. It's for precisely this reason that we're referring to them as biobanks," says Eduardo Rocha, CNRS scientist and Head of the Microbial Evolutionary Genomics Unit at the Institut Pasteur. Several thousand anti-phage cassettes are believed to exist, which means that integrons represent a huge reservoir of genes used to tackle these viruses.
The scientists also sought to characterize these anti-phage systems. They revealed sixteen functional anti-phage systems in Vibrio cholerae and E. coli that had previously been unknown. These systems are relatively small, no doubt restricted by the lower recombination rate of large cassettes. In one of these systems, the scientists even discovered the smallest currently known protein conferring phage resistance. Moreover, using models predicting the structure of proteins involved in these systems, they identified crossovers with other defense molecules. The role of these proteins is to stop bacterial growth and induce cell death to prevent phage multiplication. These functionalities were then demonstrated experimentally for newly described anti-phage systems.
What future therapies can we expect for tackling pathogenic bacteria?
These results pave the way for new biotechnological tools. For instance, the study could prompt developments to improve protection against phage infection for bacteria used in industrial processes. It also provides the basis for new avenues of research. "We're currently carrying out laboratory investigations of integron-mediated phage resistance mechanisms. As yet, only very preliminary findings have been made. It's not impossible that the difference in nature between antibiotics and phages is reflected in different bacterial resistance mechanisms," points out Céline Loot. In a more general sense, she believes that this study "does not challenge the notion of using phages against pathogenic bacteria", since "all therapeutic approaches are inevitably faced with problems in terms of resistance evolution."
Given the inevitability of the evolution process, it seems that the best strategy of tackling bacterial resistance lies in adopting multiple approaches. This is a position endorsed by Frédérique Le Roux, a scientist at the University of Montreal, who comments: "A combined approach is indeed likely to prove most effective." Didier Mazel adds: "For instance, it's possible to imagine a treatment against pathogenic bacteria that contains antibiotics combined with phage cocktails. The CRISPR approach could also be used. A few years ago, we also developed a strategy which involved inducing the expression of toxins in pathogenic bacteria by harnessing their ability to exchange genes using relatively small DNA sequences known as plasmids. A number of promising alternatives are available."
To summarize, while this work provides a fresh illustration of the incredible adaptive abilities that bacteria have at their disposal, scientists are by no means deterred in their efforts to tackle resistance.
Sedentary chromosomal integrons as biobanks of bacterial anti-phage defense systems, Science, May 8, 2025
Baptiste Darracq1,2†, Eloi Littner2,3,4†, Manon Brunie1,2, Julia Bos1, Pierre Alexandre Kaminski1, Florence Depardieu5, Weronika Slesak1, Kevin Debatisse1, Marie Touchon3, Aude Bernheim6, David Bikard5, Frédérique Le Roux7*, Didier Mazel1*, Eduardo P.C. Rocha3‡* and Céline Loot1‡*
1Institut Pasteur, Université Paris Cité, Unité Plasticité du Génome Bactérien, CNRS, UMR3525, 75724 Paris, France.
2Sorbonne Université, Collège doctoral, F-75005, Paris, France.
3Institut Pasteur, Université Paris Cité, Microbial Evolutionary Genomics, CNRS UMR3525, 75724 Paris, France.
4DGA CBRN Defence, 91710 Vert-le-Petit, France.
5Institut Pasteur, Université Paris Cité, Synthetic Biology, CNRS UMR3525, 75724 Paris, France.
6Institut Pasteur, Université Paris Cité, Molecular Diversity of Microbes lab, CNRS UMR3525, 75724 Paris, France.
7University of Montréal, Faculty of medicine, Department of microbiology, infectiology and immunology, Montreal, QC H3T 1J4, Canada.
†These authors contributed equally to this work
‡ These authors contributed equally to this work
*Corresponding authors